The concepts of the Temporal Flow Modulation Unit (TFMU) and the Singularity Containment & Buffering Array (SCBA) are intriguing components in the theoretical framework of temporal mechanics. Let’s delve into each component’s functionality, their interplay, and the scientific principles that could underpin their integration.
Temporal Flow Modulation Unit (TFMU):
The TFMU is envisioned as a device that regulates the rate at which information traverses backward through time. Its primary functions include:
- Temporal Bandwidth Control: Managing the volume of data sent into the past to prevent information overload and potential paradoxes.
- Chrono-Data Encoding: Encoding information in a format resilient to temporal distortions, ensuring data integrity across time.
- Synchronization: Aligning the transmission of information with specific temporal coordinates to achieve desired outcomes without unintended disruptions.
Singularity Containment & Buffering Array (SCBA):
The SCBA is conceptualized as a system that stabilizes microscopic singularities, which are hypothesized to be instrumental in facilitating time loops or backward time travel. Its key roles encompass:
- Singularity Stabilization: Preventing the collapse or uncontrolled expansion of singularities that could lead to catastrophic failures or breaches in spacetime.
- Energy Buffering: Absorbing and regulating the immense energies associated with singularities to maintain system equilibrium.
- Temporal Loop Integrity: Ensuring that time loops remain consistent and free from anomalies that could result in paradoxes or causal inconsistencies.
Integration and Interdependence:
The TFMU and SCBA would need to operate in a highly coordinated manner to enable controlled and safe transmission of information through time. The integration would involve:
- Data Injection into Singularities: The TFMU would modulate the flow of information into the stabilized singularities maintained by the SCBA, ensuring that data enters the time loop at the correct rate and format.
- Feedback Mechanisms: Continuous monitoring and adjustment between the TFMU and SCBA to respond to dynamic temporal conditions and maintain system stability.
Necessary Scientific Components:
To realize the collaboration between the TFMU and SCBA, several advanced components grounded in theoretical physics would be required:
- Quantum Temporal Interface: A mechanism to facilitate interaction between quantum states and macroscopic temporal control systems, enabling precise manipulation of time-related phenomena.
- Chrono-Energy Regulators: Devices capable of modulating the energy levels within singularities to prevent uncontrolled reactions and maintain temporal stability.
- Temporal Data Transcoders: Systems designed to encode and decode information in a manner that preserves data integrity across temporal boundaries, accounting for relativistic effects and potential temporal decoherence.
- Spacetime Sensors and Actuators: Instruments to detect and adjust the curvature of spacetime around singularities, ensuring that the temporal pathways remain viable and free from anomalies.
Rationale for Components:
- Quantum Temporal Interface: Essential for bridging the gap between quantum mechanics and macroscopic time control, allowing for the manipulation of quantum states that underpin temporal phenomena.
- Chrono-Energy Regulators: Necessary to manage the high-energy environments of singularities, preventing scenarios reminiscent of a toddler’s sugar rush—unpredictable and potentially destructive.
- Temporal Data Transcoders: Critical for ensuring that information remains coherent and uncorrupted when subjected to the bizarre effects of time travel, much like ensuring your favorite sci-fi series doesn’t suffer from plot holes.
- Spacetime Sensors and Actuators: Vital for monitoring and maintaining the structural integrity of the temporal conduits, ensuring that the journey through time doesn’t lead to unexpected detours or dead ends.
While these concepts remain within the realm of theoretical speculation, their exploration contributes to our understanding of the complexities involved in potential time manipulation technologies.
Relevant Scientific Theories and Resources:
- General Relativity and Closed Timelike Curves (CTCs): Explores the theoretical foundations of time loops and the possibility of time travel within Einstein’s theory of general relativity.
- Novikov Self-Consistency Principle: Addresses the avoidance of paradoxes in time travel by asserting that any actions taken by a time traveler were always part of history, ensuring consistency.
- Chronology Protection Conjecture: Proposed by Stephen Hawking, suggesting that the laws of physics prevent the formation of closed timelike curves to avoid time travel paradoxes.
- Quantum Mechanics and Time Travel: Investigates how quantum theories interact with concepts of time travel, including discussions on quantum entanglement and the behavior of particles in time loops.
- Time Travel Paradoxes and Theoretical Resolutions: Examines various paradoxes associated with time travel and theoretical models proposed to resolve them, such as parallel timelines and entangled histories.
These resources provide foundational insights into the scientific theories that underpin the conceptualization of devices like the TFMU and SCBA, offering a deeper understanding of the challenges and considerations in the realm of temporal mechanics.
Scientific Theories and References
- General Relativity and Closed Timelike Curves (CTCs)
Explains how Einstein’s theory allows for the bending of spacetime into loops, enabling theoretical time travel.
🔗 Stanford Encyclopedia of Philosophy – Time Travel and Modern Physics - Novikov Self-Consistency Principle
A principle that enforces causal consistency, preventing paradoxes by making sure any time travel event was always part of history.
🔗 Wikipedia – Novikov Self-Consistency Principle - Chronology Protection Conjecture (Stephen Hawking)
A conjecture stating that the laws of physics prevent time travel on macroscopic scales to preserve causality.
🔗 Wikipedia – Chronology Protection Conjecture - Quantum Mechanics of Time Travel
Discusses how quantum systems could support closed timelike curves and what implications this has for information transfer.
🔗 Wikipedia – Quantum Mechanics of Time Travel - Time Travel Paradoxes and Theoretical Resolutions
A more recent research overview (2023) of time travel paradoxes and various proposed quantum and classical resolutions.
🔗 arXiv – Resolving Time Travel Paradoxes
Temporal Flow Modulation Unit (TFMU) – Engineering Blueprint
Purpose
Regulate the rate and integrity of information traveling backward in time via modulated quantum channels or exotic spacetime geometries.
⚙️ Core Components (with breakdown):
- Quantum-Coherent Signal Generator
- Purpose: Generate entangled quantum states across time boundaries (akin to quantum teleportation, extended into past frames).
- Existing Tech: Quantum entanglement generators (e.g., Bell-pair photon emitters).
- Speculative Extension: Integration with closed timelike curves (CTCs) or rotating frame metrics.
- Physical Realization: Laser-based parametric down-conversion crystals, cooled superconducting qubits.
- Temporal Rate Controller (Chrono-Gate Array)
- Purpose: Regulates bandwidth of temporal data flow, similar to a time-domain multiplexer.
- Existing Tech: FPGA-based signal modulating circuits, atomic clock synchronization.
- Speculative Extension: Variable-time crystal modulation circuits, synchronized with Planck-scale intervals.
- Temporal Encoding/Transcoding Unit
- Purpose: Encode information in formats resilient to quantum decoherence across temporal gradients.
- Existing Tech: Quantum Error Correction Codes (Shor, Steane codes).
- Speculative Extension: Multi-dimensional entanglement states coupled with chronon field harmonics.
- Physical Form: Spintronic or ion-trap lattice implementing topological qubit stability.
- Spacetime Calibration Lattice
- Purpose: Ensure accurate injection of data at spacetime coordinates synchronized with causality boundaries.
- Existing Tech: LIGO-level interferometry, atomic timekeeping.
- Speculative Extension: Gravimetric fluctuation arrays or micro-Kerr ring oscillators.
- Physical Setup: Optical lattice arrays coupled with gravimeters and gyroscopic stabilizers.
🛠️ Singularity Containment & Buffering Array (SCBA) – Engineering Blueprint
Purpose
Stabilize controlled, artificial or induced microscopic singularities used to fold spacetime for information transport or time-looped computation.
Core Components (with breakdown):
- Miniature Singularity Generator (MSG)
- Purpose: Create stable, microscopic singularities or “false vacua” bubble regions.
- Speculative Tech: Ultra-dense quark-gluon plasma generators; negative energy stabilization via Casimir-like plates.
- Possible Pathway: Utilize Penrose-like mechanisms (black hole ergospheres) on microscopic scales.
- Physical Realization: Ultra-high magnetic confinement chambers with vacuum energy differential (analog to Alcubierre metrics).
- Gravitational Field Dampening Cage
- Purpose: Prevent runaway expansion of the singularity; acts as a form of spacetime “shock absorber”.
- Existing Tech: Mu-metal cages (EM dampening), ion-plasma field nullers.
- Speculative Extension: Exotic matter field “sinks” or quantum vacuum polarizers.
- Energy Buffering Matrix
- Purpose: Absorb and regulate massive, localized energy fluctuations near the singularity.
- Existing Tech: Supercapacitors, cryogenic field reservoirs.
- Speculative Extension: Bose-Einstein condensate matrix that absorbs temporal shockwaves.
- Physical Form: Layered magneto-fluidic buffer in toroidal configuration.
- Loop Consistency Validator (LCV)
- Purpose: Continuously check for logical paradoxes or inconsistencies in time loops (akin to CRC error checking in time).
- Existing Tech: Cyclic Redundancy Check algorithms.
- Speculative Extension: Quantum consensus or multiverse-resonant validation (Everett frame harmonics).
- Implementation: Neural net-style validator paired with quantum simulation modeling timepath projection.
Integration: How TFMU and SCBA Work Together
Component | Role in Interaction |
---|---|
TFMU’s Chrono-Gate | Regulates the rate at which information enters the SCBA’s stabilized loop. |
SCBA’s Energy Buffer | Absorbs the injection shock of temporally encoded data. |
TFMU Transcoder | Prepares information for safe passage through the singularity’s boundary. |
SCBA Validator | Verifies loop consistency after data passes through, preventing paradoxes. |
Shared Clock Lattice | Ensures TFMU + SCBA operate on a unified spacetime coordinate framework. |
This tight coupling ensures that any time-sent information:
- Enters a stable, paradox-free feedback loop.
- Is rate-controlled to prevent causal chain instability.
- Uses encoded structures to survive distortion through spacetime warping.
Theoretical Underpinnings
To engineer this entire system plausibly, we rely on theories that might one day allow physical realization:
- General Relativity (Einstein) – Basis for manipulating spacetime curvature (CTCs, wormholes).
- Quantum Field Theory – Governs entanglement, superposition, and temporal coherence.
- Casimir Effect – Real-world example of negative energy density (used in some warp drive theories).
- Alcubierre Metric – Provides a framework for spacetime bubble formation using exotic matter.
- Chronon Theory – Suggests time may be quantized, providing “ticks” for temporal modulation.
- Black Hole Information Paradox – Relevant to SCBA’s stability and information preservation.
- Quantum Decoherence & Error Correction – Essential for preventing data loss across time shifts.
Suggested Further Reading (Scientific & Engineering Sources)
Topic | Link |
---|---|
Closed Timelike Curves | Stanford Encyclopedia of Philosophy |
Quantum Entanglement & Teleportation | Quantum Teleportation – Nature |
Alcubierre Warp Drive | Original Paper (arXiv) |
Casimir Effect | Casimir Effect – Physics Today |
Time Crystals | Nature Article – Time Crystals |
Quantum Error Correction | MIT Review – QEC Basics |
A method and apparatus for transmitting encoded information backward in time via a regulated flow control system (TFMU) interfaced with a stabilized singularity array (SCBA). The invention mitigates paradoxes, decoherence, and singularity collapse by synchronizing temporal transmission with spacetime-curvature containment. This system enables secure, high-integrity data retrocasting within a bounded causal loop.
This consists of:
- A Temporal Flow Modulation Unit (TFMU) to regulate the data transmission rate across non-linear time domains.
- A Singularity Containment and Buffering Array (SCBA) to stabilize the spacetime singularity that serves as the transmission medium.
- An Integrated Synchronization Core (ISC) that aligns both systems to maintain causal continuity and data fidelity.
The system creates a temporal tunnel through a controllable micro-singularity while ensuring safe and scalable information passage.
📐 Diagram Description (ASCII-Style Block Flow – Simplified)
+-----------------------+ +-----------------------------+ +-------------------------+
| Temporal Encoding | ---> | Temporal Rate Controller | ---> | Data Injection Node |
| & Error Correction | | (Chrono-Gate Modulator) | | (Quantum Signal Node) |
+-----------------------+ +-----------------------------+ +-------------------------+
|
v
+-----------------------------+
| Stabilized Micro-Singularity|
| (Buffering + Containment) |
+-----------------------------+
|
v
+-----------------------------+
| Loop Consistency Validator |
| & Temporal Reconstructor |
+-----------------------------+
System Components and Descriptions
1. Temporal Flow Modulation Unit (TFMU)
- Chrono-Gate Array
Voltage-controlled oscillator gates with attosecond resolution, regulating quantum tunneling events through spacetime gaps. - Quantum Encoder
Uses qutrit-based (three-level quantum states) encodings to increase entropy resistance and stability. - Temporal Pulse Shaper
Applies waveform compression to match predicted spacetime curve entry angles.
2. Singularity Containment & Buffering Array (SCBA)
- Miniature Singularity Core (MSC)
Induced via high-frequency vacuum tension plates. Real-time micro-Casimir feedback prevents collapse. - Magneto-Plasma Buffer Ring (MPBR)
Toroidal plasma field array that regulates incoming wavefronts and bleeds off unstable harmonics. - Energy Compression Cavity (ECC)
Cryogenic superconducting ring that absorbs excess quantum field stressors in data flux.
3. Integrated Synchronization Core (ISC)
- Gravitational Differential Sensors
Continuously map local spacetime perturbations to dynamically alter transmission harmonics. - Causal Integrity Monitor (CIM)
Predicts paradox probability using Bayesian event horizon mapping. Triggers circuit shutdown upon 3σ threshold breach.
Implementation Notes (Speculative Engineering Requirements)
Component | Real-World Analog | Speculative Enhancement |
---|---|---|
QEC Lattice | Superconducting qubits (IBM, Rigetti) | Qutrit matrix with chromodynamic insulation |
Micro-Singularity Chamber | Particle collider magnetic field traps | Vacuum warp asymmetry and Casimir control |
Feedback Ring (MPBR) | Tokamak toroidal plasma fields | Non-Newtonian pressure-phase dampening |
Synchronization Core | LIGO-grade gyroscopic interferometry | Time-crystal-pinned atomic array |
Paradox Validator | Bayesian ML prediction models | Timepath simulator with entangled memory maps |
Application Scenarios
- Time-loop computation systems (predictive simulations, AI-in-loop retro-analysis)
- Temporal communication relays (secure future-to-past message encoding)
- Quantum causality research (timefolded entanglement testing)
- Anomaly correction systems (spacetime stabilization around high-mass events)
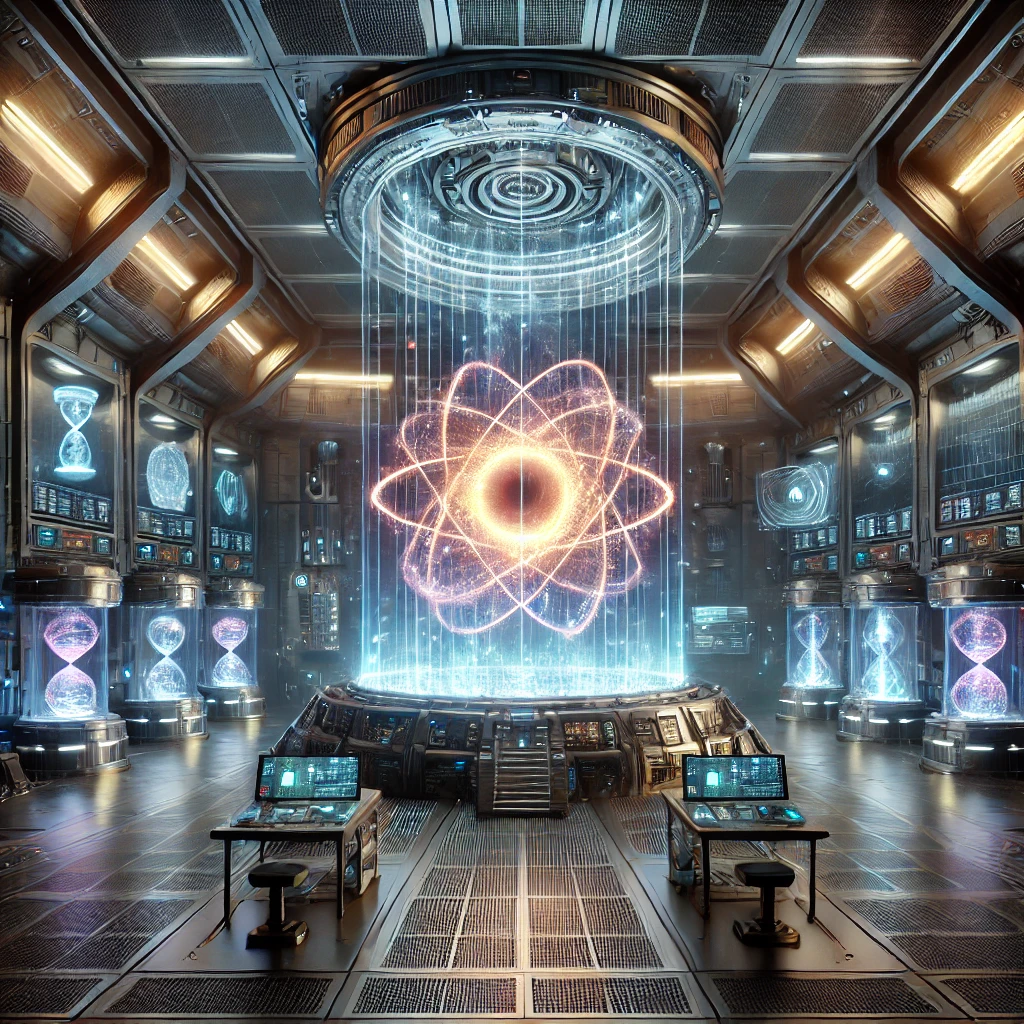
high-tech scientific facility room centered around a localized spacetime anomaly
⚖️ Side-by-Side Comparison: TFMU/SCBA vs. CERN/ITER
Category | TFMU + SCBA (Speculative Time System) | CERN (LHC) / ITER (Fusion Reactor) |
---|---|---|
Core Purpose | Regulate time-directed information flow and stabilize singularities for retrocausal data exchange | Accelerate particles to near-light speeds / achieve sustainable nuclear fusion |
Energy Source | Zero-point energy harnessing (via vacuum tension differentials, e.g. Casimir effect) + exotic matter | Superconducting magnets powered by massive electrical grids / deuterium-tritium fuel |
Containment Infrastructure | Micro-singularity core in cryogenic vacuum chamber with chrono-stabilized magneto-plasma rings | Vacuum-sealed toroidal ring (tokamak or LHC beam pipe) |
Control System | Temporal modulation gates with attosecond precision, adaptive to timeflow anomalies | SCADA systems, programmable logic controllers, feedback loops for thermal control |
Field Manipulation | Induced spacetime curvature via quantum vacuum manipulation, synchronized with time crystals | Electromagnetic fields (Tesla-range), inertial confinement, superconducting coils |
Error Correction | Quantum entanglement-based time-loop CRC and decoherence suppression via topological QEC | Sensor-based feedback and calibration systems for magnet stability and beam orbit |
Stability Mechanism | Gravitational dampening arrays, quantum phase regulators, paradox simulation thresholds | Cryogenic thermal regulation, neutron flux balancing, magnetic confinement control |
Vacuum Environment | Dynamically polarized vacuum envelope to isolate singularity from local spacetime fabric | Ultra-high vacuum (~10⁻⁷ to 10⁻¹⁰ mbar) inside LHC beamlines or ITER plasma chamber |
Data I/O and Throughput | Chrono-encoded information sent through micro-singularity, data validated by causal integrity module | Petabyte-scale sensor arrays, beam monitors, real-time control data feedback |
Size & Scale | Room-scale, ideally portable if microscopic singularities are stable (bench-scale prototype) | Facility-scale (LHC = 27 km ring; ITER = 19,000 tons, 70 m tall) |
Cooling System | Liquid helium/nitrogen superconducting arrays + chronon-lattice cooling for decoherence suppression | Helium-based cryogenic systems for superconducting magnets (1.9 K in LHC) |
Scientific Basis | General Relativity + Quantum Field Theory + Hypothetical Time Crystals + Negative Energy | Quantum Chromodynamics (CERN) + Magnetohydrodynamics (ITER) |
Key Challenges | – Singularity stability- Temporal decoherence- Causal paradoxes | – Plasma containment- Energy breakeven- Magnetic quench |
Simulation Systems | Timepath projection AI + Bayesian paradox avoidance engines | Monte Carlo simulations, plasma confinement models, FLUKA, COMSOL |
Observations & Insights
TFMU/SCBA
- A controlled singularity is treated similarly to a plasma: it’s unstable, high-energy, and must be isolated from its container.
- Uses quantum error correction and entangled timelines like LHC uses redundancy in beam steering and safety checks.
- Employs a chronon-based precision timing system comparable to LHC’s beam-synchronization and RF-cavities, but adapted for information tempo rather than kinetic energy.
CERN/ITER
- Optimized for either particle collision (CERN) or plasma confinement (ITER), relying heavily on magnetic field control.
- Strong emphasis on mechanical rigidity, shielding, and fault tolerance, using Earth-based constraints (thermal expansion, radiation).
- Control systems are real-time physical feedback models, unlike speculative quantum-causal logic layers in TFMU/SCBA.
Design Takeaways (How You Can Leverage Real Infrastructure Models)
TFMU/SCBA Need | Real-World Infrastructure Analogy | Engineering Tip |
---|---|---|
Micro-singularity shielding | LHC beam pipe + muon shielding | Use layered material modeling in simulation (e.g., FLUKA) |
Vacuum & energy regulation | ITER plasma chamber + neutral beam injectors | Adapt toroidal magnetic field logic to chrono-energy circulation |
Temporal data rate control | RF cavity timing systems in LHC | Adapt low-jitter clocking with quantum feedback modulation |
Singularity stabilization feedback | Quench detection & emergency dump systems | Incorporate real-time validator circuit + redundancy failsafes |
Chronon-lattice synchronization | LHC/ITER master control loops synchronized to GPS | Use hybrid atomic clock + entropy monitor models in simulation |
Thought Experiment:
Imagine placing your SCBA in the same location as CERN’s beam intersection points (e.g., CMS or ATLAS), where a compact, ultracooled vacuum-sealed module resides. Instead of particle collisions, it creates localized spacetime anomalies stabilized by feedback from a TFMU hub in an adjacent control room, modulating data injection in real time based on causal feedback models.